There are five major mechanisms
of crustal thickening that need to be considered (Bird, 1984). Some
of these mechanisms may affect this system to a greater degree than
others. The first mechanism is Sedimentation. Estimates for sediment
volumes from the Cretaceous to the present spread over the Great Plains
would yield a crustal thickness of only 200 m which is just a few percent
of the total crustal thickness increase. The second mechanism is magmatic
intrusion. This effect is estimated to contribute just 0.1% of total
crustal thickness in the Rocky Mountain foreland. Furthermore, vast
undiscovered plutons in the lower crust would be required to provide
even a mild contribution to the overall crust in the area (Bird, 1984).
The third mechanism is crustal shortening which is easily quantified
by measuring displacement across compressional faults. This does explain
a small amount of the thickening in the Rocky Mountains but is absent
in the Great Plains.
Of these five models, the last two are favored for the Rocky Mountain
foreland and Great Plains. Both rely on displacement of material
in the lower crust. The fourth mechanism is lateral shear in the
lower crust. Simply, this would be described by flow in a channel
driven by a plate edge, like a piston in a tube. And finally, the
fifth mechanism is passive flow, or less formally oozing.
Shearing and Pushing
Bird (1984) strongly advocated this mechanism of crustal thickening.
As the name implies, this mechanism requires that a slab is moving
below the Western US providing the basal shear stress. More specifically,
it requires that the Farallon plate underwent flat subduction and
was at a significantly shallow angle of subduction, such that the
top of the slab was in contact with the lower crust of the continent.
Whether or not the Farallon slab underwent flat subduction is still
under heavy debate. In the figure below, we can see that the continental
crust was under ridden by the Farallon slab for over 1000 km before
the slab took on a steeper angle and formed a hinge line. It’s
intuitive that the larger the distance between the edge of the continental
plate and this hinge line, the less likely that it’s physically
accurate.
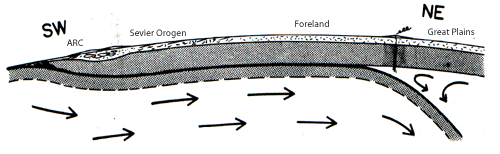
From Bird 1984 paper, showing importance of the direct under riding
of the Farralon plate all the way to the Great Plains.
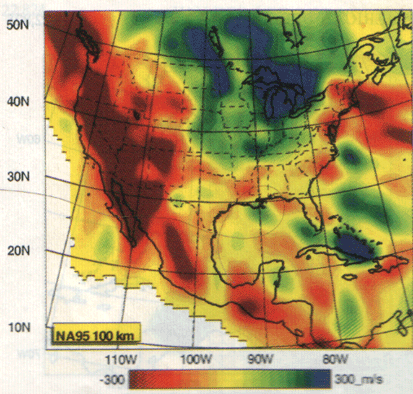
This image of a tomography model by van der Lee (1997) helps
illustrate some of the cause for doubt about flat subduction. The
relatively low velocity at the depth where a flat slab should be
raises doubt. Also, other evidence from volcanism and a paucity
of similar subduction geometries casts down on the reliability
of this model.
Still, even if flat subduction did not occur, some sort of subduction
did occur. So at some level, the lower crust near the edge of the
continental crust was pushed to the east. Whether the hingeline is
close to the plate boundary or not, lower crust was pushed eastward
and influences the following mechanism.
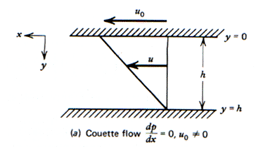
This image shows the basic concept of basal shear crustal flow (Turcotte and Schubert, 2002).
Oozing
This model is best visualized through a simple diagram. The model
below is from a paper by Clark and Royden (2000) dealing with similar
thickening mechanisms for the margin of Tibet. This mechanism is
also referred to as channel flow or Poiseuille flow.
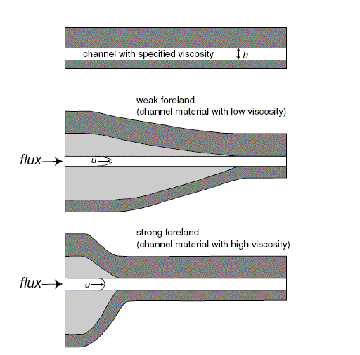
The different steepness of the two lower images is dependent on the
viscosity of the lower crust (Clark and Royden, 2000).
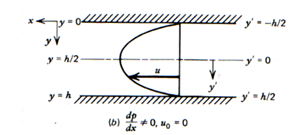
This image shows how the flow works in a channel such as the lower
crust (Turcotte and Schubert, 2002).
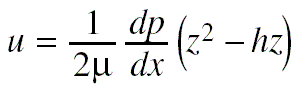 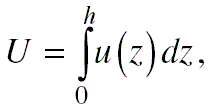 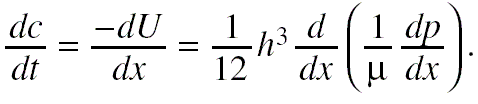
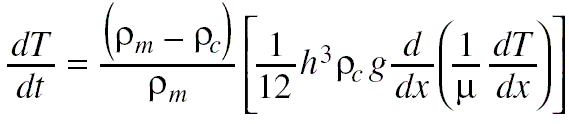
These equations describe the mathematics
of channel flow. Where µ is
the viscosity, h is the thickness of the channel p is the
pressure and z is the depth and T(x) is the topographic elevation.
In
this last equation, rhoc and rhom are fairly well constrained
and the
value that is determined is really h³/µ. With viscosity
determined from other methods, this gives good constraints on
the whether
or not this model is really valid.
A shortcoming of favoring this type of support is that it is considered
to be reversible. After all, if it could flow to the East so easily
from the Colorado Plateau, there would be little keeping it from
flowing further to the East, North or South. If we return to our
plot of crustal thickness for North America we’ll see that
this accommodation space is not so easily found. Overall this mechanism
of crustal thickening seems to hold water pretty well.
Effects on viscosity
From measurements made after observing
postglacial rebound, dynamic viscosity values of 4*10^19 Pa s. Deeper mantle
values have also been determined as being about 10^21 Pa s. From this, µ (viscosity)
can be determined from the simple relationship, dynamic viscosity = µ/density.
It is also important to know the temperature dependence of viscosity.
µ (t) = µ *exp(E/RT)
where µ is the viscosity, E is a diffusion coefficient parameter, R is
the universal gas constant and T is the temperature. This basically
tells us that viscosity will vary significantly in the low temperature range.
Aside from composition, this temperature dependence will affect when
lower crust would move (if at all). Also, as shown above, the shape of the
wedge will also be affected by the viscosity value.
|